PHPP10 brings changes to how the ground temperatures are calculated in the ground sheet that Passive House designers should familiarise themselves with. The short version is PHPP10 will generate more accurate results—ie its predictions will better match reality—especially for lightweight constructions with less insulation between the building and the ground.
What does it mean for hitting the Passive House target? The more accurate ground methodology and not shifting the seasons to account for the northern/southern hemisphere will increase heating demand for the same design, compared to the old way of figuring it, by about 0.8kWh/m2/year for our temperate climates and lightweight constructions. The exact amount will depend on the individual design and its climate. This is not insignificant given the 15kWh/m2/year maximum for certification and will require compensation—either by adding extra insulation in the slab or somewhere else in the building.
Critically, if your project was designed in PHPP9, you do not need to convert that project to PHPP10. It is legitimate to certify the building on the basis of the calculations submitted in PHPP9. Do not open yourself up to a power of trouble by upgrading your calculations to PHPP10. Needless to say, for every new project you begin, work in PHPP10.
Benefits
The revised methodology in PHPP10 is an improvement over PHPP9 in two main ways.
- First and foremost, it will more accurately predict heat loss in high-performance buildings because for temperate climates, it is no longer necessary to choose between two temperatures on the basis of when heating and cooling begins, as PHPP10 calculates one integrated heat flow.
- The ground heat flow calculation can better handle ground coupling and will more accurately calculate cooling benefits and thermal mass buffering of poorly-insulated slabs.
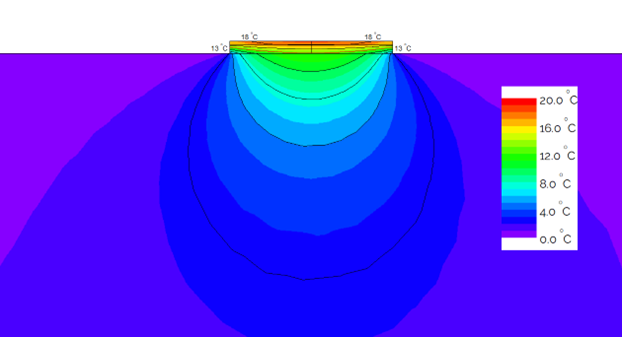
Illustration of the heat lens below an insulated slab in New Zealand assuming adiabatic slab edges. Calculated using Flixo to the ISO10211 standard. This heat lens is primarily a function of the slab area-to-perimeter ratio and slab insulation levels. PHPP10 estimates a very similar heat lens to account for the benefit of the ground conductivity as well as the dynamic benefit on the heating season energy consumption and cooling season reduction in overheating.
The issues with PHPP9 ground sheet calculations
In PHPP9 the ground sheet calculated the monthly average ground temperatures for use in the monthly method. PHPP assumes that the room temperature in summer is higher than in winter. As the calculation is performed using a constant room temperature, two results are returned for the equivalent soil temperatures under the floor slab for each month! One is for the winter indoor temperature, the other for the summer indoor temperature. However, it is a self-evident truth of physics that the ground can only be one temperature at any one moment. Thus PHPP9 has to select one of the two temperatures or use an interpolation for each month.
This could lead to problems though, because determining whether a month falls in the heating or the cooling period is not entirely simple. Inaccuracies could arise in particular in temperate climates like ours and for lightweight buildings where there is less insulation to ground because higher room temperatures can heat up the ground and reduce the heat loss in the winter. We could see this in play when comparing PHPP9 groundsheet results to a full dynamic ground simulation.
How it’s changed
The methodology in PHPP10 has been revised to include the seasonal variation in room temperatures. It directly outputs the heat flow into the ground for each month.
The global change in PHPP10 that means designers don’t have to use a clumsy workaround for Southern Hemisphere projects and also makes ground sheet calculations more accurate. The PHPP9 workaround just flipped the months, for example February in the north became August in the south. But it didn’t take into account that February has 28 days, whereas August has 31. There were two consequences. The extra three days resulted in a longer heating period. The heat losses from February (which are calculated from U*A*delta_T*28 days), become bigger in August but the solar gains were calculated from the monthly solar radiation sum and remain unchanged, so the daily heating demand increases.
For light-weight New Zealand constructions (mostly built from timber), these combined to result in a longer heating period compared to heavyweight constructions (like European buildings made of concrete and brick). The difference in ground heat losses between PHPP9 and 10 methodologies becomes more relevant in the shoulder seasons. If the New Zealand example buildings had been heavyweight construction, the difference between PHPP9 and 10 would have been very small. This is why the European experts at IPHA didn’t think the workaround caused any issues. We had to point out to them the impact it caused for New Zealand’s typical construction styles and climate.